As the plane began its descent into Medford, we dropped into the blanket of smoke that covered southwestern Oregon and northern California. It was late July 2018, and several major fires were burning in the region. I was en route to join a Cal Fire (California Department of Forestry and Fire Protection) team investigating a fatal incident that had taken place two days earlier. What the group leader told me over the phone had sent chills up my spine: “A firefighter has been killed in a fire tornado. His vehicle was thrown hundreds of feet across the ground.”
I, perhaps more than anyone, had known that this might happen someday. Ten years earlier I had gotten my first look at the aftermath of a fire tornado. The object, almost 1,000 feet in diameter, had moved out of the Indians Fire in California and overrun a group of firefighters. So strong was the wind that trying to get to safety felt like running through chest-deep water, one of the survivors told me. Fortunately, the men were standing on a paved two-lane highway, which probably saved their lives: had they been even 10 feet away and among the trees and grass, they would have died. When I reached the site, massive oak branches lay all around, and the ground had been scoured of pebbles.
The scene left me impressed and worried. A fire tornado could evidently harm firefighters taking refuge in areas usually thought to be safe. It had been a close call. Many of us had seen fire whirls, dust-devil-sized rotating columns of fire, and did not regard them as particularly dangerous. In contrast, fire tornadoes—which combine the destructive power of fire with that of winds as ferocious as in an actual tornado—were so rare as to be almost mythical. Even I, a firefighter since 1996 and a fire-behavior researcher for eight years, had heard of only one, from a story a veteran firefighter told me.
On supporting science journalism
If you're enjoying this article, consider supporting our award-winning journalism by subscribing. By purchasing a subscription you are helping to ensure the future of impactful stories about the discoveries and ideas shaping our world today.
On returning to my home base at the Missoula Fire Sciences Laboratory in Montana, I conducted a literature survey. It turned up reports, most rather sketchy, of several fire tornadoes that had occurred around the world in the near and distant past. So scant was the information on the subject that scientists did not even agree on what qualified as a fire tornado. Massive forest fires can generate so-called pyrocumulonimbus (pyroCb) clouds at high altitudes. These are ice-capped thunderclouds that condense from the moisture released above a fire—from the vegetation it consumed, from the water vapor in the atmosphere and as a by-product of combustion itself. A few researchers held that only those fire vortices that connect to overhead pyroCb clouds are true fire tornadoes. By that definition, only one had ever been documented, in a 2003 firestorm near Canberra, Australia. It had left a damage path almost 15 miles long.
That framework seemed far too restrictive to be of much use to firefighters, however. Using the working definition of a fire tornado as a fire whirl with tornadolike wind speeds, my colleague Bret Butler and I had gathered up whatever documentation we could find and consolidated it into firefighter-training manuals and classes. But now I found myself driving south toward the Carr Fire just outside Redding, Calif., to investigate the death of a firefighter in a fire tornado—a tragedy I had long sought to avert.
The Carr Fire Tornado
The site looked like a war zone. Neither the famous tornado researcher Josh Wurman, whom I had recruited for the investigation, nor I had ever seen anything like this. Entire blocks of homes had been leveled, with only the foundations remaining. Roofing and other debris littered the area, and vehicles had been rolled multiple times over the ground. Trees were uprooted or broken off, and flying particles of sand and rock had stripped them of their bark. Three power-line towers built of metal lattice, each roughly 100 feet tall, had been blown down, with one of them having been lifted off its base and carried 1,000 feet through the air. A 40-foot shipping container had been torn apart, and a steel pipe was wrapped around downed power poles.
We estimated that the winds could have reached 165 miles per hour, a speed that occurs in class 3 tornadoes on the Enhanced Fujita scale. (This scale rates tornadoes on a scale ranging from 0 to 5, with 5 indicating the fastest and most destructive winds.) In California, only two regular tornadoes of this strength had ever been recorded. Peak temperatures of the burning gases inside the fire tornado may have reached almost 2,700 degrees Fahrenheit. The object was more than 1,000 feet wide at its base and, according to radar imagery, three miles high. It lasted for at least 40 minutes, during which time it moved slowly across the ground, leaving a path of destruction nearly a mile long.
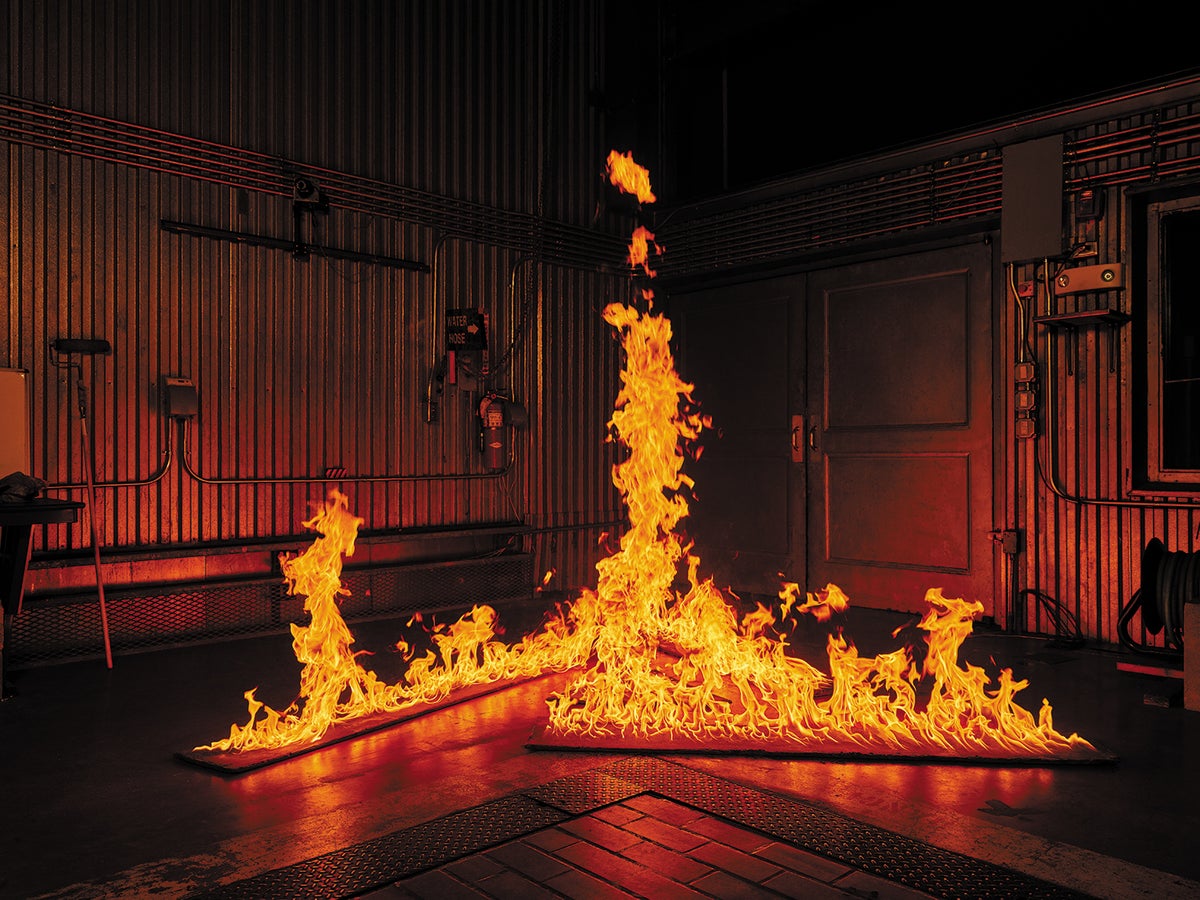
Burning boards arranged in a rough triangle allow air to swirl into the central area, where another fire gathers the rotation into a vortex. Forest or urban fires of certain shapes can similarly generate fire tornadoes.
Credit: Spencer Lowell
Our team interviewed witnesses and collected video evidence in the hope of learning from the event. The fire tornado occurred on the evening of July 26, 2018, in the course of a forest fire covering thousands of acres northwest of Redding. So extensive and intense was the fire that it generated pyroCb clouds at altitudes higher than three miles. Suddenly, at around 5:30 P.M., the flames raced eastward, killing firefighting bulldozer operator Don Smith, as well as a civilian in his home. As the wildfire neared the outskirts of Redding, it spawned a number of fire whirls and threw embers more than a mile ahead of the fire and across the Sacramento River. These started several “spot,” or small, isolated fires near two subdivisions at the end of a dead-end road. An extremely chaotic scene unfolded as firefighters tried to evacuate homeowners and save houses even as their escape route was being cut off. People were literally running for their lives.
Redding firefighter Jeremy Stoke headed to the scene to help. Just as he was arriving, at about 7:30 P.M., the fire tornado formed over the road, trapping residents and firefighters at the subdivisions. It apparently caught Stoke on the road. He transmitted a mayday call on his radio before powerful winds rolled his truck multiple times; it eventually came to rest against a tree hundreds of feet away. Stoke was found hours later, dead from traumatic injuries.
Two Cal Fire vehicles being driven down the road had most of their windows blown out and were battered by flying debris. Strangely, one of the trucks was damaged mostly on the driver’s side and the other on the passenger side—even though they were only 150 feet apart and facing the same direction—indicating the rotating motion of the air. The occupants huddled on the floorboards to save themselves from projectiles. Three nearby bulldozers also had their windows blown out, with one operator getting glass in his eye and another receiving serious burns to his hands. A retired police officer who was driving out realized his truck bed was on fire and pulled over; he survived but sustained burns to his airways. Most tragically, on the outer edge of the revolving inferno two children and their great-grandmother perished inside their burned home.
In the Laboratory
What can we learn from an event like this? Can we predict when and where a fire tornado will occur so that we can evacuate residents and firefighters? What causes fire tornadoes? A first step toward answering these questions is to look back in history. In 1871 a town in Wisconsin was devastated by what was probably a fire tornado, judging by the massive amount of debris—which included a house—thrown around. In 1964 the Polo Fire in California spawned one that injured four people and destroyed two homes, a barn, three cars and an avocado orchard. One of the most horrific occurred during the World War II incendiary bombing of Hamburg, Germany: the resulting firestorm generated a fire tornado that, according to geographer Charles Ebert, was up to two miles wide and three miles tall. More than 40,000 civilians died in the conflagration.
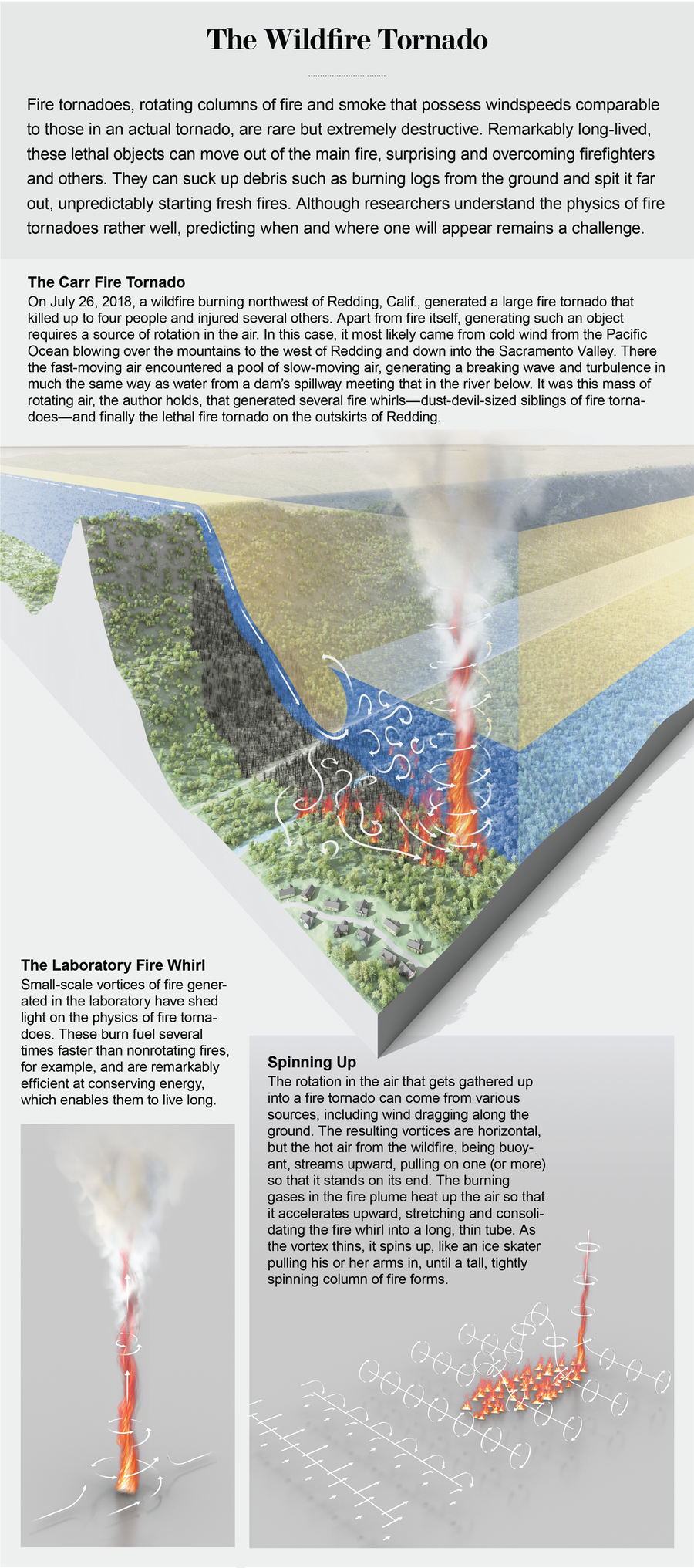
Credit: Bryan Christie Design
In 1923 a major earthquake sparked an urban fire in Tokyo. As it spread from building to building, residents evacuated to an open area between the structures. A large fire tornado formed over this area, killing an estimated 38,000 people in 15 minutes. For more than half a century the accepted explanation for this terrible event was that a regular tornado happened to form at the exact same time and location as the fire. But in the 1980s and 1990s engineers S. Soma and K. Saito of the University of Kentucky used historical records to construct a small-scale model of the actual fire, painstakingly reproducing its geometry and ambient winds. Their laboratory fire generated a vortex—proving that the original one was not a coincidence but was caused by the fire itself.
This research built on pioneering lab work conducted two decades earlier, when George Byram and Robert Martin of the U.S. Forest Service Southern Research Station created small fire whirls at their facility in Macon, Ga. Their apparatus consisted of a small circular pool of burning alcohol surrounded by cylindrical walls with vertical slits, which forced drafts into the fire to enter in a rotating motion. Significantly, the resulting fire whirl caused the fuel to burn—and its energy to be released—up to three times faster than in a nonrotating fire. The rotating wind appears to have increased the rate of burning by pushing the flames down toward the surface of the alcohol, heating it up. Subsequent research has found the energy-release rate to be enhanced by up to seven times in such fires.
Something similar occurs in wildfire whirls and fire tornadoes. A heated piece of wood generates hundreds of different flammable gases, the further combustion of which yields flames. The strong horizontal, rotating winds in the fire tornado can force the flames down into the vegetation, causing it to burn more fiercely.
In 1967 Howard Emmons and Shuh-Jing Ying of Harvard University surrounded a stationary lab fire with a cylindrical wire screen that could be spun at various speeds, imparting rotation to the air flowing into the flames. The researchers measured the wind velocity and temperature distribution of the fire whirl thus generated, getting a glimpse into its inner workings. They found that, apart from fire itself, the formation of such a vortex requires a source of rotation and a mechanism to intensify it.
A fire tornado has essentially the same hydrodynamics. Significant vorticity often exists in the atmosphere—generated by wind curling around mountains or dragging along the ground or by variations in density and pressure. The fire itself carries out two other crucial functions: it concentrates the rotation and stands it up, so that a tight tube of air ends up spinning around a vertical axis.
First the hot air rising above the fire pulls in replacement air at the base, thereby gathering rotating air from the surroundings. Some of the vorticity might originally be around a horizontal axis, but once air is sucked up into the fire plume, its hot, buoyant upward stream causes the axis to tilt to a vertical orientation. Second, although the upwardly moving air starts out slow when it is near the ground, it heats up as the gases in it burn. The air pressure all around the vortex forces the hot, light air within the core upward. The accelerating air in the fire plume stretches the fire whirl or fire tornado vertically along its axis, reducing its diameter, much as pulling apart a clump of dough causes a long, thin neck to form. The reduced diameter drives the air to turn faster to conserve its angular momentum—the same effect seen when a spinning ice skater draws in his or her arms.
It appears that when a fire whirl or fire tornado moves over a burning area, it stretches to a considerable height and spins tight and fast, but when it moves over an already burned area, it spreads out and slows down into a diffuse cylinder of smoke. Sometimes the rotating object is so wide and slow that firefighters fail to perceive it. The direction of motion of the vortex across the ground depends on ambient winds and details of terrain in ways that we have yet to understand.
Emmons and Ying also found that fire vortices are remarkably efficient at conserving their rotational energy, which makes them (unfortunately) rather long-lived. The Indians Fire tornado, for example, lasted for about an hour. As the fire tornado spins up, two opposing forces in the radial direction strengthen: centrifugal force pulling a parcel of rotating air outward and, in opposition, low pressure in the core pulling it inward. The resulting balance limits the movement of air in the radial direction and therefore the loss of energy from the vortex. In contrast, nonrotating fires exchange roughly 10 times more energy with the surrounding atmosphere. This mechanism also makes fire whirls thinner and taller than nonrotating fires because with practically no air being drawn in, except at the base, less oxygen is available for combustion. Thus, some of the fuel gases must travel high up the core before they encounter sufficient oxygen to burn.
Just as dangerous, the towering column of hot, low-density gases induces very low pressure at the base of the whirl. Drag near the ground slows the rotation, reducing the centrifugal force pushing the air outward. Because the inward force generated by pressure remains the same, however, the wind near the ground streams into the fire tornado. It ends up acting like a giant vacuum cleaner, sucking air and, often, burning debris into the base, forcing it vertically up the core at extreme velocities and spitting it out from high up—unpredictably generating spot fires.
In the Field
Despite all this knowledge about the physics of fire tornadoes, we still cannot predict where and when one will occur. One thing is clear, however: given how rare fire tornadoes are even though a large, intensely burning fire always has the capacity to concentrate rotation, the essential factor for their appearance seems to be the presence of a strong source of rotation.
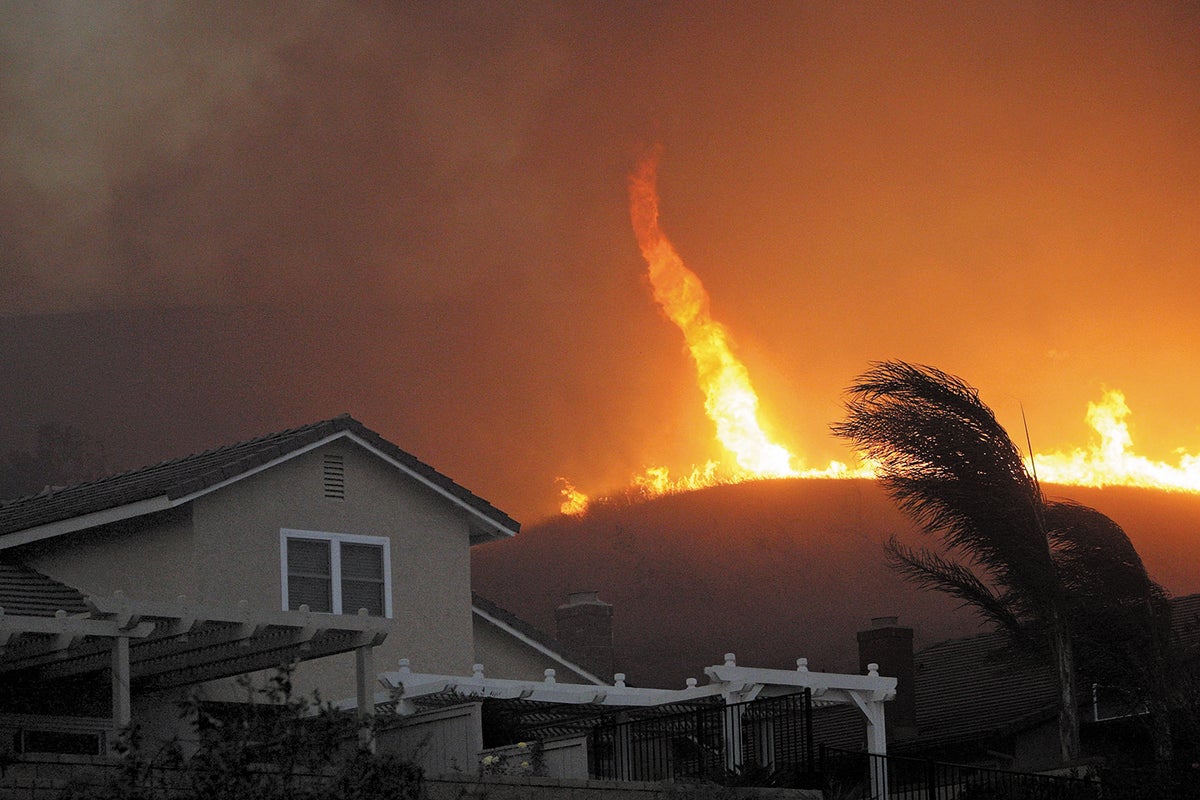
Corona fire in Yorba Linda, Calif., in November 2008 generated a flaming vortex—possibly a fire tornado—that threatened homes.
Credit: David McNew Getty Images
We know from case studies, for example, that one of the likeliest locations for fire tornadoes to form is on the lee side of a mountain. Wind blowing around the mountain causes swirling motions on the downwind side, like water moving around a large rock in a river. A fire burning there can gather and stretch this rotation into a fire tornado. But matters are in fact more complicated: Fiery vortices can also show up on flat ground and in calm wind conditions. For example, a large fire whirl in Kansas was likely generated by a cold front that collided with warm ambient air as it passed over a fire in a field. And a 2007 study by Rui Zhou and Zi-Niu Wu of Tsinghua University in Beijing showed that multiple fires burning in certain specific configurations—which can happen when a fire throws embers ahead of itself, starting new fires—may even generate their own rotation by inducing jets of air to flow along the ground between them.
So where did the rotation that caused the deadly Carr Fire tornado come from? Given the several fire whirls that preceded the fire tornado, an abnormally high amount of rotation obviously existed in the area. On a hunch, I asked Natalie Wagenbrenner, a colleague at the Missoula Fire Sciences Laboratory, to run some specialized computer simulations of the weather that day. Her studies showed that cool, dense air from the Pacific Ocean was being pushed eastward and over the top of a mountain range west of Redding. This cool air was much heavier than the hot air in the Sacramento Valley: the Redding airport reported a peak temperature that day of 113 degrees F, a record. So gravity caused the air to accelerate as it moved down the slopes toward the valley, much like water flowing downhill. Oddly, these strong surface winds stopped abruptly—right where the fire tornado formed.
What happened to the wind? Finally, I realized that a hydraulic jump was occurring—the atmospheric equivalent of what happens to water when it flows down the spillway below a dam. When the fast-moving water hits the low-speed pool below, the surface of the water jumps upward, forming a breaking wave that stays in place and marks the boundary between the two flows. This region contains intense swirling motions. In much the same way, the cold, dense air speeding down the mountainside hit the slow-moving pool of air in the Sacramento Valley, most likely generating the powerful rotation that formed the Carr Fire tornado [see graphic above]. N. P. Lareau of the University of Nevada and his colleagues speculated in a 2018 paper that the pyroCb clouds overhead, which reached altitudes of up to seven miles even as the fire tornado formed, helped to stretch the vortex to a great height, thereby thinning it and spinning it up even more.
If wildfires continue to become more extensive, we may encounter such lethal objects more frequently. The silver lining is that lessons learned from studying them carefully might help prevent future tragedies. I am hopeful that further research into fire tornadoes, combined with advances in weather prediction and computing power, will, in the near future, give us the ability to issue fire tornado warnings—possibly saving lives.
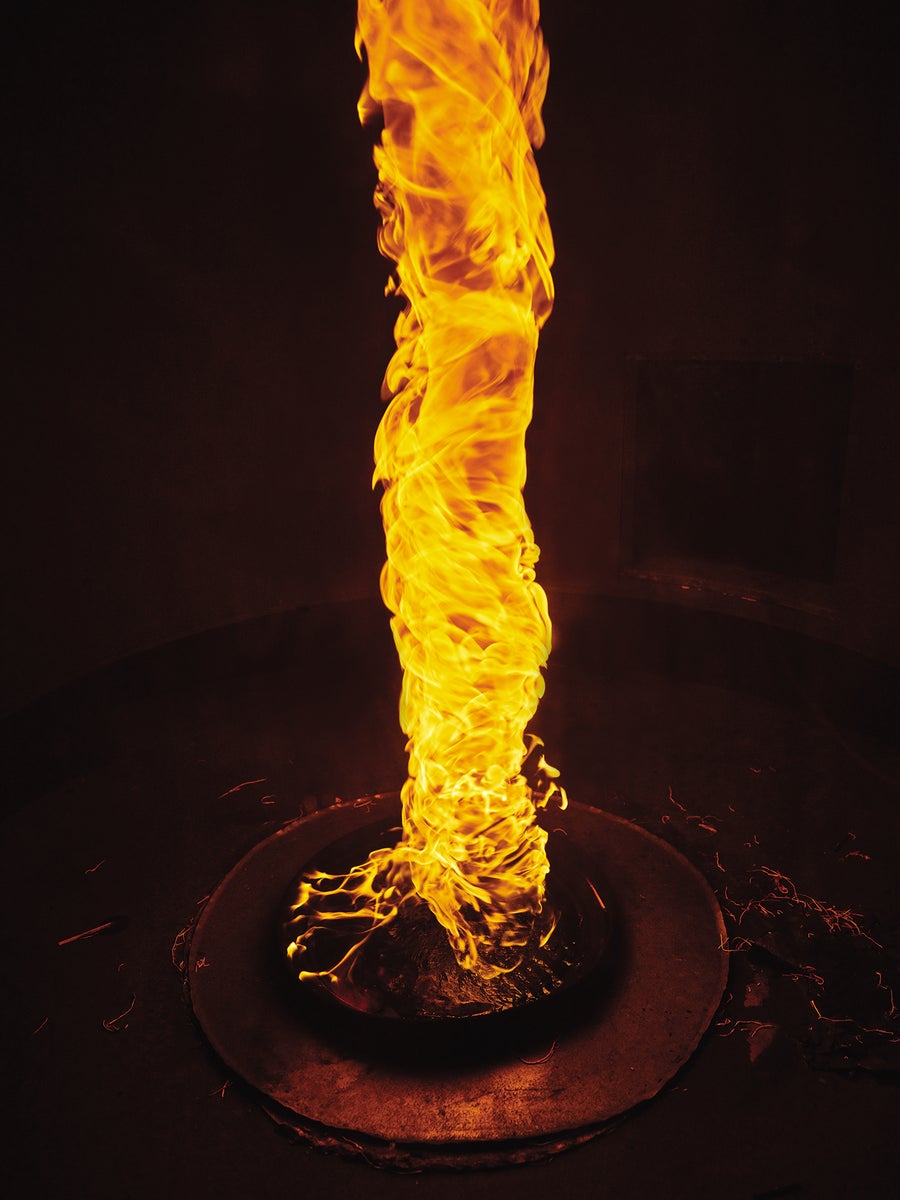
Fire whirl is formed by rotating air drafting into a pan of burning alcohol at the Missoula Fire Sciences Laboratory in Montana. Credit: Spencer Lowell